Grid growth and decarbonization: An unhappy couple
The advent of AI training and inference applications, combined with the continued expansion of the digital world and electrification of the economy, raises two questions about electricity generation capacity: where will the new energy be sourced from, and how can it be decarbonized?
Groups, such as the International Energy Agency and the Electric Power Research Institute, project that data center energy demand will rise at an annual rate of 5% or more in many markets over the next decade. When combined with mandated decarbonization and electrification initiatives, electricity generation capacity will need to expand between two and three times by 2050 to meet this demand.
Many data center operators have promoted the need to add only carbon-free generation assets to increase capacity. This is going to be challenging: deployment of meaningful carbon-free generation, including energy from geothermal sources and small nuclear reactors (SMRs), and battery capacity, such as long duration energy storage (LDES), are at least five or more years away. Given the current state of technology development and deployment of manufacturing capacity, it will likely take at least 10 years before they are widely used on the electricity grid in most regions. This means that natural gas generation assets will have to be included in the grid expansion to maintain grid reliability.
Impact of wind / solar on grid reliability
To demonstrate the point, consider the following example. Figure 1 depicts the current generation capacity in Germany under five different weather and time of day scenarios (labeled as scenario A1 to A5). Table 1 provides the real-time capacity factors for the wind, solar and battery assets used for these scenarios. German energy demand in summer is 65 GW (not shown) and 78 GW (shown by the dotted line) in winter. The blue line is the total available generation capacity.
Figure 1. Grid generation capacity under different weather and time of day scenarios
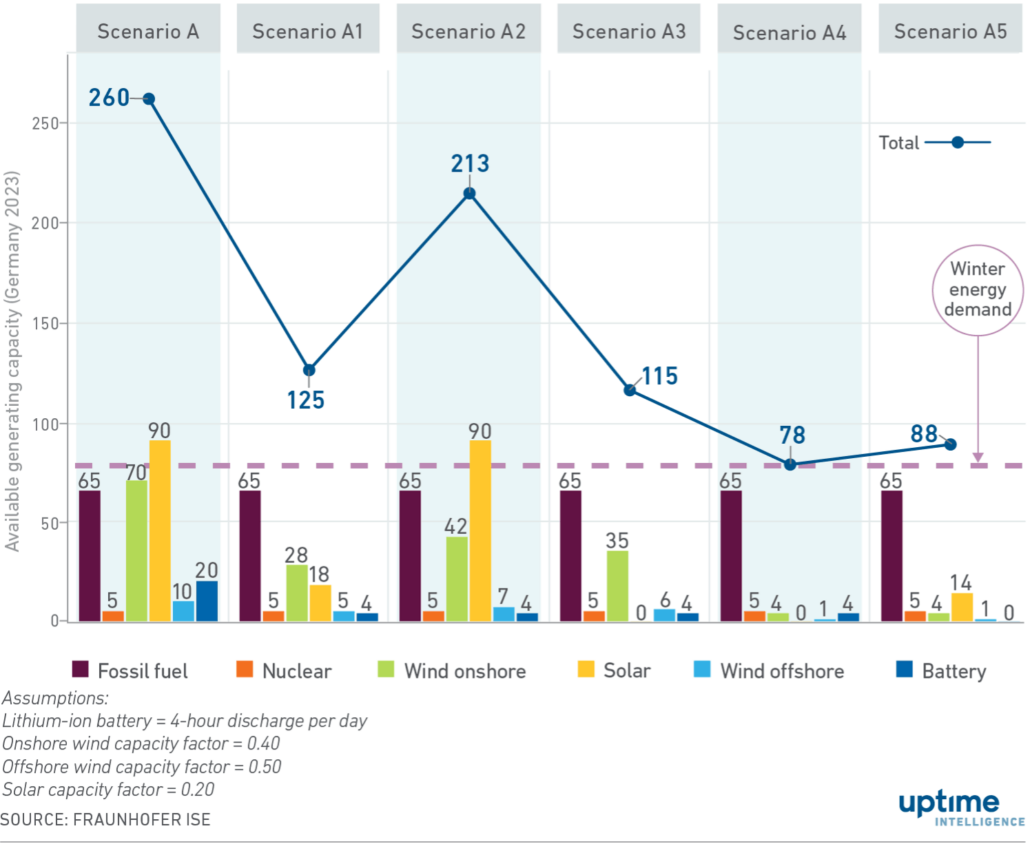
Table 1. Generation capacity factors for scenarios A and B
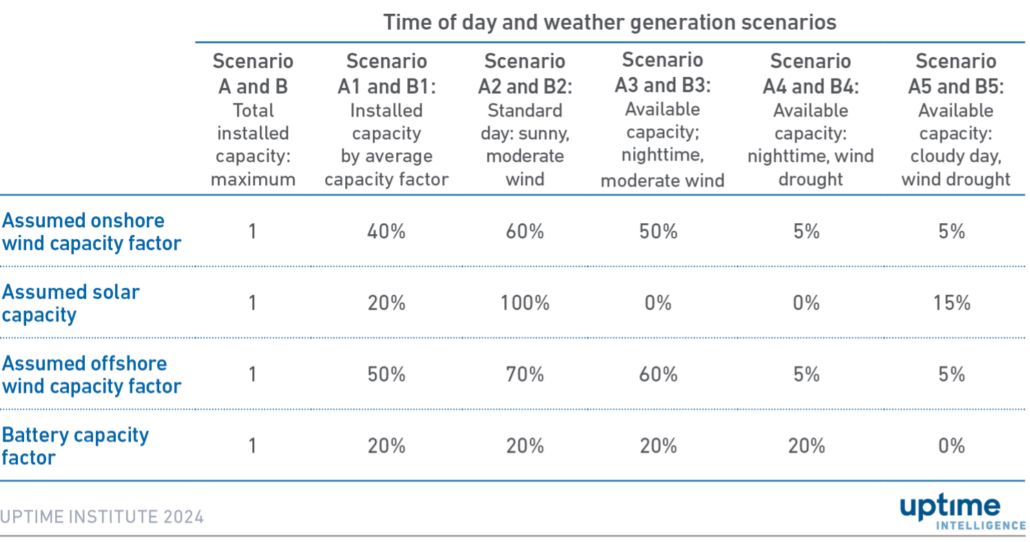
Scenario A details the total available generation capacity of the German electricity grid in 2023. The grid has sufficient dispatchable generation to maintain grid reliability. It also has enough grid interconnects to import and export electricity production to address the over- or under-production due to the variable output of the wind and solar generation assets. An example of energy importation is the 5 GW of nuclear generation capacity, which comes from France.
Scenario A1 depicts the available generation capacity based on the average capacity factors of the different generation types. Fossil fuel and nuclear units typically have a 95% or greater capacity factor because they are only offline for minimal periods during scheduled maintenance. Wind and solar assets have much lower average capacity factors (see Table 1) due to the vagaries of the weather. Lithium-ion batteries have limited capacity because they discharge for two to four hours and a typical charge / discharge cycle is one day. As a result, the average available capacity for Germany is only half of the installed capacity.
Grid stress
The average capacity only tells half the story because the output from wind, solar and battery energy sources vary between zero and maximum capacity based on weather conditions and battery charge / discharge cycles.
- Scenarios A2 and A3 illustrate daytime and nighttime situations with high solar and wind output. In these scenarios, the available generation capacity significantly exceeds electricity demand.
- In scenario A2, the 139 GW of available wind and solar assets enable Germany to operate on 100% carbon-free energy, export energy to other grid regions and charge battery systems. Fossil fuel units will be placed in their minimal operating condition to minimize output but ensure availability as solar assets ramp down production in the evening. Some solar and wind-generating assets will likely have to be curtailed (disconnected from the grid) to maintain grid stability.
- In scenario A3, the wind and fossil fuel assets provide sufficient generation capacity to match the demand. The output of the fossil fuel assets can be adjusted as the wind output modulates to maintain grid balance and reliability. Discharging the batteries and importing or exporting electricity to other grid regions are unnecessary.
- Scenarios A4 and A5 show the challenges posed by solar and wind generation variability and limited battery discharge times (current lithium-ion battery technologies have a four-hour discharge limit). These scenarios represent the 10th to 20th percentile of wind and solar generation asset availability.
Low wind and low or nonexistent solar output push the available generation capacity close to the electricity demand. If several fossil fuel assets are offline and/or imports are limited, the grid authority will have to rely on demand management capacity, battery capacity and available imports to keep the grid balanced and avoid rolling blackouts (one- to two-hour shutdowns of electricity in one or more grid sub-regions).
Demand management
The scenario described above is not peculiar to Germany. Wind and solar generation assets represent the bulk of new capacity to meet new data center and electrification demand and replace retiring fossil fuel and nuclear assets as they are (1) mandated by many state, province and national governments and (2) the only economically viable form of carbon-free electricity generation. Unfortunately, their variable output leaves significant supply gaps of hours, days and seasonally that cannot currently be addressed with carbon-free generation assets. These gaps will have to be filled with a combination of new and existing fossil fuel assets, existing nuclear assets (new plants, as new conventional or small modular nuclear reactors are eight years or more in the future) and demand management.
As the installed solar and wind generation capacity increases to a greater percentage of supply capacity, data center operators will play a major role in demand management strategies. Several strategies are available to the utilities / data center operators, but each has its drawbacks.
Backup generation systems
Significantly, emergency generator sets will need to be utilized to take data centers off the grid to address generation capacity shortfalls. This strategy is being deployed in the US (California), Ireland and other data center markets. In conversations with operators, several report being requested by their grid authority to deploy their emergency generators to relieve grid demand and ensure stability during the summer of 2023.
As new data centers are built and deployed, operators in stressed grid regions (i.e., those with a high percentage of capacity delivered by wind and solar assets) should plan and permit their emergency generators to be operated for 25% or more of the year to support grid reliability in the face of variable wind and solar generation asset production.
Workload reduction
Demand management can also be achieved by reducing data center energy consumption. Google has reported the development of protocols to shut down non-critical workloads, such as controllable batch jobs, or shift a workload to one or more data centers in other grid regions. The reports did not provide the volume of workloads moved or the demand reductions, which suggests that they were not significant. These tools are likely only used on workloads controlled by Google, such as search workloads or work on development servers. They are unlikely to be deployed on client cloud workloads because many IT operators are uncomfortable with unnecessarily stressing their operations and processes.
An example of an IT enterprise operation that could support demand management is a financial organization running twin data centers in two different grid regions. When grid stability is threatened, it could execute its emergency processes to move all workloads to a single data center. In addition to receiving a payment for reducing demand, this would be an opportunity to test and validate its emergency workload transfer processes. While there is a strong logic to justify this choice, IT managers will likely be hesitant to agree to this approach.
Outages and reliability problems are more likely to emerge when operational changes are being made and demand management payments from the grid authority or energy retailer will not compensate the risk of penalties under service level agreements. The use of backup generators will likely be the preferred response, though problems, such as issues with starting and synchronizing generators or transfer switch failures, can be experienced when switching to generators.
New solar and wind capacity needed
The energy demands of new data centers and the broader electrification of the economy will require the installation of new electricity generation capacity in grid regions around the world. Large colocation and cloud service providers have been particularly vocal that these generation assets should be carbon-free and not involve new fossil fuel generation assets. An analysis of the impact of increasing the wind, solar and battery generation capacity on the German grid by 20% to support a 15% increase in demand reveals the inherent dangers of this position.
Figure 2 details the available grid generation capacity under the five available capacity scenarios (see Table 1) when the wind, solar and battery capacities are increased by 20%. This increase in generating capacity is assumed to support a 15% rise in energy demand, which increases winter demand to 90 GW.
Figure 2. Impact of a 20% increase in wind and solar generation
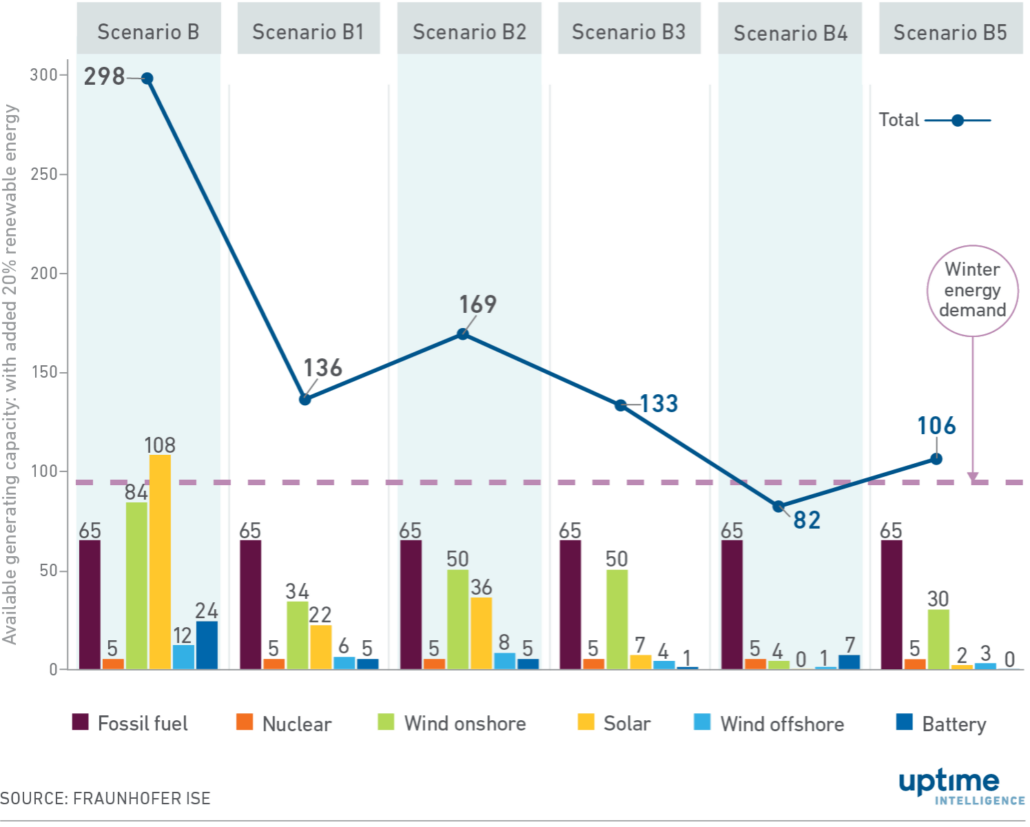
The 20% generating capacity increase delivers sufficient available capacity for periods of moderate to high wind and solar capacity (scenarios B2 and B3). There is a sufficient capacity reserve (about 10% to 15% of demand) to provide the needed generation capacity if some fossil fuel-based generators are offline or the imports of nuclear-generated electricity are not available.
However, the capacity increase does not significantly increase the available capacity at periods of low solar and wind output, putting grid stability at risk in scenarios B4 and B5. The available capacity in scenario B4 increases by only 4 GW, which is insufficient to meet the new demand or provide any capacity reserve. Under scenario B5, there is barely enough capacity to provide a sufficient reserve (about 10% to 15% of capacity). In both cases, grid stability is at risk and some combination of demand management, battery capacity and imports will be required.
Until reliable, dispatchable carbon-free electricity technologies, such as SMRs, geothermal generation and LDES, are developed and deployed at scale, grid stability will depend on the presence of sufficient fossil fuel generation assets to match energy supply to demand. The deployed capacity will likely have to be 75% to 100% of projected demand to address three- to 10-day periods of low solar and wind output and expected seasonal variations in wind and solar production.
To enable growth and ensure reliable electricity service while increasing renewable energy generation capacity, data center operators will need to balance and likely compromise their location selection criteria, decarbonization goals, and the size of their electrical demand growth.
Managing growing power demand
Data center operators will have to reevaluate their sustainability objectives in light of the need to increase the overall grid generation capacity and the percentage of that capacity that is carbon-free generation while maintaining grid stability and reliability. Operators should consider the following options to take meaningful steps to further decarbonize the grid:
- Set realistic goals for global operations to consume 80% to 90% carbon-free energy over the next decade rather than making unrealistic near-term net-zero claims. Studies have shown significant technical and economic obstacles to decarbonizing the last 10% to 20% of electricity generation while maintaining grid reliability. Most data centers currently operate on 10% to 50% (or less) carbon-free energy. Working to achieve 80% to 90% consumption of carbon-free energy by 2035 at an enterprise level is a reasonable goal that will help to support the grid’s decarbonization.
- Support research, development and deployment of reliable, dispatchable carbon-free electricity generation and LDES technologies. Many large data center operators are making exemplary efforts in this area by supporting innovative, on-site operational tests and entering into prospective contracts for future purchases of energy or equipment.
- Design, permit and deploy backup generation systems for 2,000 to 3,000 hours of annual operation to support grid reliability during periods of low production from wind and solar generation assets. Operators are already activating these systems to manage grid demands in some jurisdictions, such as Ireland and California in the US, that have a large installed wind or solar generation base with high variability in day-to-day and seasonal output.
- Intensify efforts to improve the efficiency of the IT infrastructure. Efforts to improve IT efficiency need to include the deployment of server power management functions and storage capacity optimization methods; increases in the utilization of installed server, storage and network capacity to higher levels; refreshing and consolidating IT equipment; and designing energy efficiency into software applications and algorithms with the same intensity and commitment given to providing performance and function. The IT infrastructure consumes 70% to 90% of the energy in most data centers yet data shows that more than 60% of IT operators largely ignore the opportunities to drive IT equipment efficiency improvements.
- Operators need to improve and validate techniques to move workloads from a data center in a stressed grid region to an unstressed grid region. Cloud workloads can be shifted to underutilized data centers in an unstressed grid region. Where a financial operator runs twin data centers, the full workload of paired, twin data centers can be moved to the facility that is in an unstressed grid. Data center operators will be hesitant to deploy these techniques because they can place critical operations at risk.
The data center industry can maintain its sustainability focus despite the expected growth in power demand. To do so, IT operators need to refocus on continually increasing the work delivered per unit of energy consumed by the IT infrastructure and the megawatt-hours of carbon-free energy consumed by data center operations. With available technologies, much can be done while developing the technologies needed to decarbonize the last 10% to 20% of the electricity grid.
The Uptime Intelligence View
Accelerating the growth of data center capacity and energy consumption does not have to imperil the industry’s drive toward sustainability. Instead, it requires that the sector pragmatically reassess its sustainability efforts. Sustainability strategies need to first intensify their focus on increased IT infrastructure utilization and work delivered per unit of energy consumed, and then take responsible steps to decarbonize the energy consumed by data centers while supporting necessary efforts to grow generation capacity on the grid and ensure grid stability.