24×7 carbon-free energy (part two): getting to 100%
Digital infrastructure operators have started to refocus their sustainability objectives on 100% 24×7 carbon-free energy (CFE) consumption: using carbon-free energy for every hour of operation.
To establish a 24×7 CFE strategy, operators must track and control CFE assets and the delivery of energy to their data centers and use procurement contracts designed to manage the factors that affect the availability and cost of renewable energy / CFE megawatt-hours (MWh).
The first Update in this two-part series, 24×7 carbon-free energy (part one): expectations and realities, focused on the challenges that data center operators face as they look to increase the share of CFE consumed in their facilities. This second report outlines the steps that operators, grid authorities, utilities and other organizations need to take to enable data centers to be 100% powered by CFE. As previously discussed, data centers that approach 100% CFE using only wind and solar generation currently have to buy several times more generation capacity than they need.
Figure 1 illustrates the economic challenges of approaching 100% 24×7 CFE. To reach 50% to 80% CFE consumption, the price premium is likely to be 5% or less in markets with high CFE penetration (30% or more of the generated MWh). The levelized cost of electricity (LCOE) is the average net present cost of the electricity based on the cost of generation over the lifetime of an individual or group of generation facilities. Beyond 80% 24×7 CFE, the electricity rate escalates because not enough CFE generation and storage assets are available to provide a reliable electricity supply during periods of low wind and solar generation.
To push toward 100% CFE consumption, operators will need to take actions — or support efforts — to increase grid region interconnects and to develop and deploy reliable, dispatchable, carbon-free generation and long duration energy storage (LDES) capacity. LDES are storage technologies that can store energy for extended time periods, discharge electricity continuously for one to 10 days or longer, and supply electricity at rates that are competitive with other generation technologies.
Figure 1. The cost of electricity as the percentage of 24×7 CFE approaches 100%
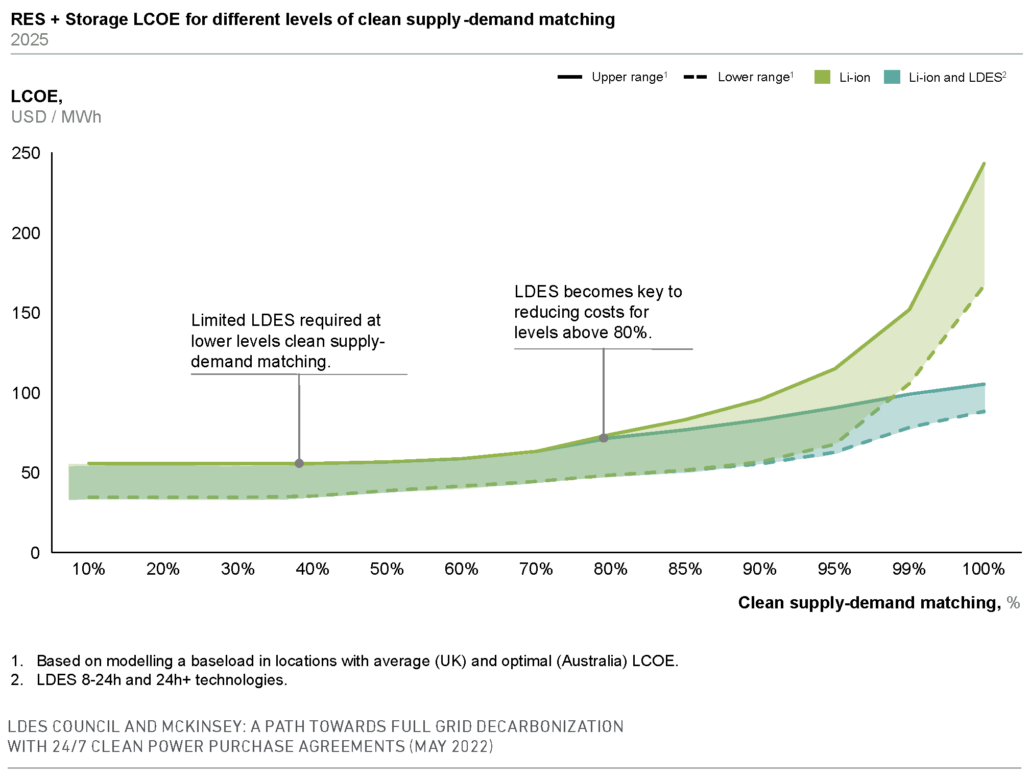
Increase grid region interconnections
Wind and / or solar resources are prevalent in some regions and absent in others. In many cases, areas with productive wind and solar resources are distant from those with high electricity demand. Connecting abundant resources with high-demand areas requires the build-out of high voltage interconnects within and between grid regions and countries. Recent news and trade articles have detailed that the current lack of grid interconnections and flexibility to support more distributed generation assets is slowing the building and deployment of planned solar and wind generation facilities. Numerous published studies detail the high voltage distribution system buildouts needed to support 100% CFE in grid regions around the globe.
The untapped potential of inter-regional grid interconnections is illustrated by the excess generation capacity associated with Google’s wind power purchase agreements (PPAs) for its Oklahoma and Iowa data centers in the Midwest region of the US, where wind generation is high. Google has four data centers in the adjacent US Southeast region (where wind generation is low). These have a low percentage of 24×7 CFE so would benefit from access to the excess Iowa and Oklahoma wind capacity. However, the extra wind capacity cannot be transported from the Midwest Reliability Organization (MRO) grid region to the Southeast Reliability Corporation (SERC) because of a lack of high-voltage interconnection capacity (Figure 2).
Figure 2. Google wind and solar assets by grid region (US)
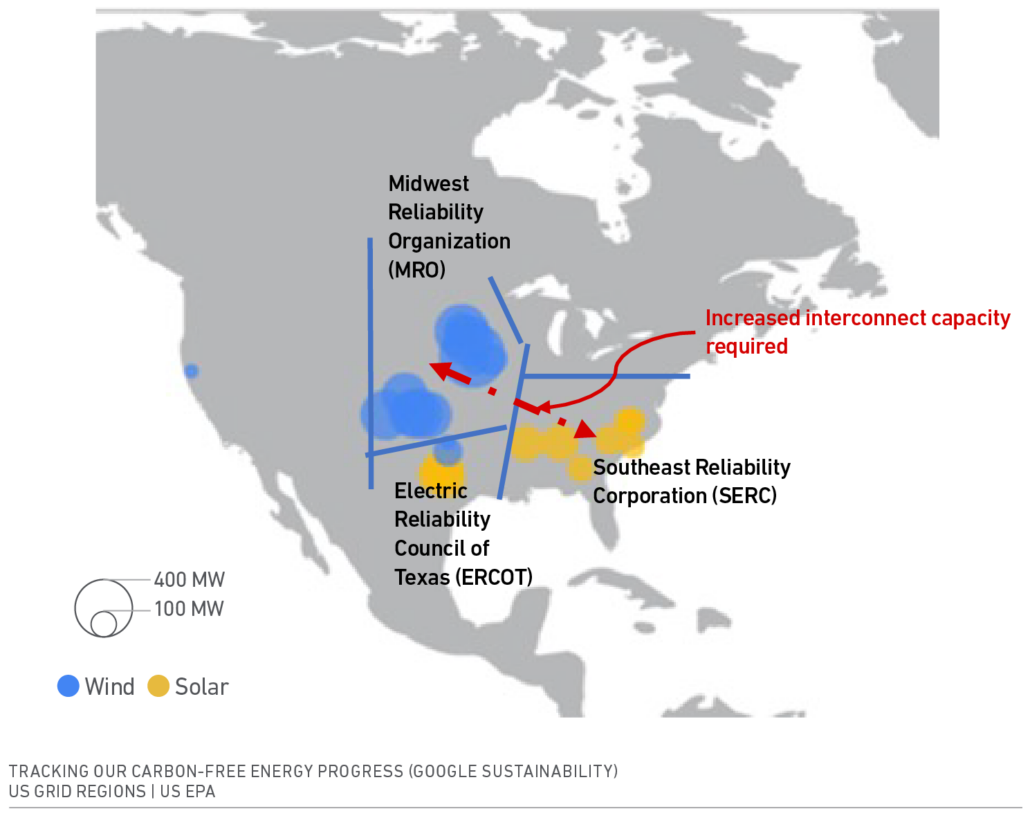
The buildout of these projects is complicated by permit issues and financing details. Again, the Google example is constructive. The Clean Line, a 2 GW (gigawatt) high voltage transmission line between MRO and SERC, was proposed by a development consortium around 2013. The line would have enabled Google and other purchasers of wind power in the MRO region to transport excess wind generation to their data centers and other facilities in the SERC region. Clean Line may have also transported excess solar power from the SERC region to the MRO region.
The permitting process extended over four years or more, slowed by legal challenges from property owners and others. The developer had difficulties securing finance, because financiers required evidence of transportation contracts from MRO to SERC, and of PPAs with energy retailers and utilities in SERC. Generators in MRO would not sign transmission contracts without a firm construction schedule. Regulated utilities, the primary energy retailers in SERC, were hesitant to sign PPAs for intermittent renewable power because they would have to hold reliable generation assets in reserve to manage output variations. The developer built a lower capacity section of the line from MRO to the western edge of SERC, but the remainder of the planned system was shelved.
Reliable CFE generation
Complete decarbonization of the electricity supply will require deploying reliable carbon-free or low-carbon energy generation across the global energy grid. Nuclear and geothermal technologies are proven and potentially financially viable, but designs must be refreshed.
Nuclear generation is undergoing a redesign, with an emphasis on small modular reactors. These designs consist of defined, repeatable modules primarily constructed in central manufacturing facilities and assembled at the production site. They are designed to provide variable power output to help the grid match generation to demand. Like other systems critical to grid decarbonization, new reactor types are in the development and demonstration stage and will not be deployed at scale for a decade or more.
Geothermal generation depends on access to the high-temperature zones underground. Opportunities for deployment are currently limited to areas such as Hawaii, where high-temperature subterranean zones are close to the surface.
Horizontal drilling technologies developed by the petroleum industry can access deeper high-temperature zones. However, the corrosive, high-pressure, and high-temperature conditions experienced by these systems present many engineering challenges. Several companies are developing and demonstrating installations that overcome these challenges.
Hydrolytic hydrogen generation is another technology that offers a means to deploy reliable electricity generation assets fueled with hydrogen. It has the advantage of providing a market for the excess wind and solar generation overcapacity discussed earlier in the report. The economics of hydrolytic systems suffer from current conversion efficiencies of 75% or less and uncertain economic conditions. Production incentives provided by the US Inflation Reduction Act, and similar programs in Japan, Australia, the EU and the UK, are attracting initial investments and accelerating the installation of hydrogen generation infrastructure to demonstrate system capabilities and reduce unit costs.
These three technologies illustrate the challenges in increasing the reliable carbon-free energy capacity available on the electricity grid. This discussion is not an exhaustive review, but an example of the technical and manufacturing challenges and extended timelines required to develop and deploy these technologies at scale.
Long duration energy storage
Long duration energy storage (LDES) is another critical technology needed to move the electricity grid to 100% 24×7 CFE. Table 1 details various battery and physical storage technologies currently being developed. The table represents a general survey of technologies and is not exhaustive.
Table 1. Long duration energy storage (LDES) technologies
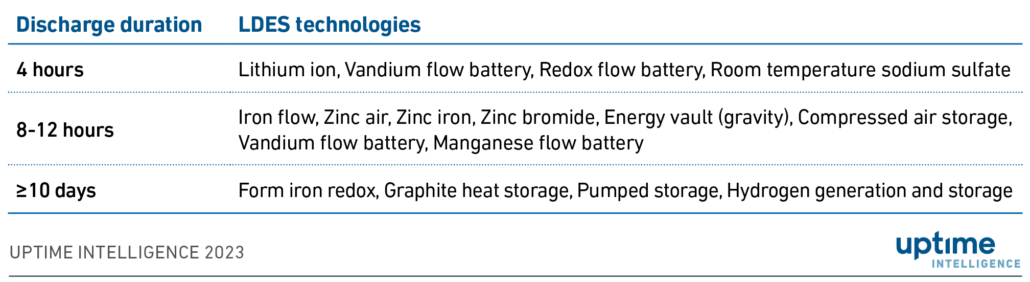
Each type of battery is designed to fill a specific operating niche, and each type is vital to attaining a low-carbon or carbon-free electricity grid. All these technologies are intended to be paired with wind and solar generation assets to produce a firm, reliable supply of CFE for a specified time duration.
- 4-hour discharge duration: Lithium-ion batteries are a proven technology in this category, but they suffer from high costs, risk of fires, and deterioration of battery capacity with time. Other technologies with lower capital costs and better operating characteristics are under development. These batteries are designed to manage the short-term minute-to-minute, hour-to-hour variations in the output of wind and solar generation assets.
- 8- to 12-hour discharge duration: These storage systems address the need for medium-duration, dispatchable power to fill in variations in wind and solar generation over periods beyond 4 hours. The batteries will provide continuous, quasi-reliable round the clock power by charging during periods of excess wind and solar production and discharging during periods of no or low MWh output. These systems will rely on sophisticated software controls to manage the charge/discharge process and to integrate the power generation with grid demand.
- ≥10 day discharge duration: These storage systems are designed to support the grid during longer periods of low generation — such as low solar output caused by several cloudy days or a multiday period of low wind output — and to cover significant week-to-week output variations. As with the 8- to 12-hour LDES systems, the energy cost and the frequency of discharge to the grid will govern the economics of the operation.
The cost of energy from the battery depends on three factors: the energy cost to charge the batteries, the round-trip efficiency of the charging / discharging process, and the number of charge / discharge cycles achieved for a given period.
The impact of the cost of power to charge the batteries is evident. The critical point is that the economics of a battery system depend on buying power during periods of grid saturation when wholesale electricity prices are approaching zero.
Roundtrip efficiency dictates the quantity of excess energy that has to be purchased to compensate for the inefficiencies of the charge / discharge cycle. If the roundtrip efficiency is 80%, the battery operator must purchase 1.25 MWh of energy for every 1 MWh delivered back to the grid. To make the economics of a battery system work, charging power needs to be purchased at a near zero rate.
To be profitable, the revenue from energy power sales must cover the cost of buying power, operating and maintaining the battery system, and paying off the loan used to finance the facility. The economics of an 8- to 12-hour battery system will be optimized if the system charges / discharges every 24 hours. If the battery system can only dispatch energy on one out of seven days, the revenue is unlikely to cover the financing costs.
Effectively integrating storage systems into the grid will require sophisticated software systems to control the charging and discharging of individual battery systems and the flow to and from battery systems at a grid level. These software systems will likely need to include economic algorithms tuned to address the financial viability of all the generating assets on the grid.
Conclusions
Transitioning the global electricity grid to CFE will be a long-term endeavor that will take several decades. Given the challenges inherent in the transition, data center operators should develop plans to increase their electricity consumption to 70% to 80% CFE, while supporting extended grid interconnections and the development and commercialization of LDES technologies and non-intermittent CFE electricity generation.
There will be grid regions where the availability of wind, solar, hydroelectric and nuclear generation assets will facilitate a faster move to economic 100% CFE. There are other regions where 100% CFE use will not be achieved for decades. Data center operators will need to accommodate these regional differences in their sustainability strategies. Because of the economic and technical challenges of attaining 100% CFE in most grid regions, it is unrealistic to expect to reach 100% CFE consumption across a multi-facility, multi-regional data center portfolio until at least 2040.
Uptime Institute advises operators to reset the timing of their net zero commitments and to revise their strategy to depend less on procuring renewable energy credits, guarantees of origin, and carbon offsets. Data center operators will achieve a better outcome if they apply their resources to promote and procure CFE for their data center. But where CFE purchases are the key to operational emissions reductions, net zero commitments will need to move out in time.
Data center managers should craft CFE procurement strategies and plans to incrementally increase CFE consumption in all IT operations — owned, colocation and cloud. By focusing net zero strategies on CFE procurement, data center managers will achieve real progress toward reducing their greenhouse gas emissions and will accelerate the transition to a low-carbon electricity grid.